KATYAYANI CHOWDHRY |
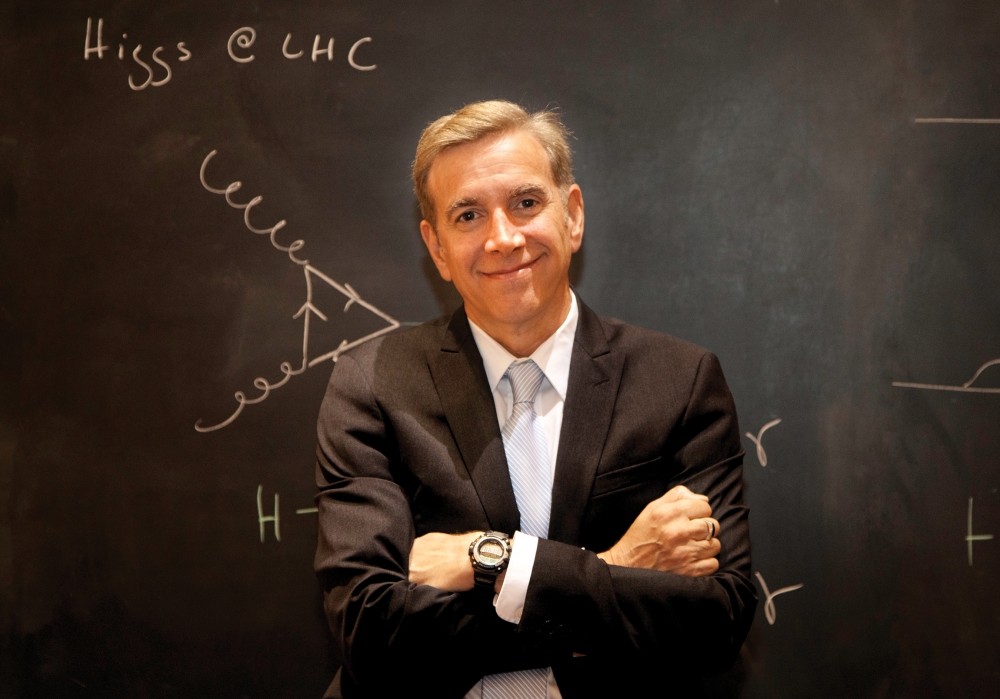
On 3rd June, 2015, CERN (European Organisation For Nuclear Research) restarted its LHC (Large Hadron Collider), after a two-year hiatus of technical upgrades, to run non-stop for the next three year period.
Located 100ms below the Franco-Swiss Border, the atom smasher runs 27km round, well- literally smashing atoms, simulating conditions of what might have been 14 billion years ago, just a few seconds after the Big Bang.
2 stable proton beams running in opposite directions at speeds of 13 TeV (teraelectronvolts), guided by superconducting magnets, collide in 4 especially arranged detectors around the circumference: ATLAS, ALICE, LHCb and CMS; in a bid to discover exotic matter that could explain the creation of the universe through its substructure, supersymmetry superpartners and shed light upon the elusive dark matter.
In 2012, the CMS (Compact Muon Selenoid) Experiment, a collaborative venture of 38 nations, 2000 Physicists across 180 institutions, made the sensational discovery of the Higgs Boson.
Dr. Joseph Incandela, Professor of Physics at UC Santa Barbara and then Spokesperson for the CMS, played a pivotal role in the particle’s discovery. He and his team (CMS-collaboration) were responsible for the detector’s design, construction and operation, software, data analysis and management.
Universally acclaimed for his invaluable contribution to Particle Physics and recently the Boson, Dr. Incandela has just been inducted to the haloed National Academy of Sciences, a significantly prestigious achievement for anyone in the scientific field.
He expressed himself as “overwhelmed, very happy and honoured” at being thus celebrated.
In an exegetical interview, the academician explains the nitty-gritty of particle physics, the future for the CMS experiment and what is popularly dubbed now as the God Particle.
Q1/ After the successful first run, what are you expecting to find this time round with the LHC?
We are going to be looking at proton-proton collisions at an energy of 13 TeV. This will be the first time above 8 TeV and the added energy opens up the potential for producing new heavy particles that we never had access to in the past. The most watched areas will be the searches for evidence of Supersymmetry (SUSY), or any strongly produced new particles that could be seen quite early. By the end of this year, we will have sensitivity to SUSY partners to the heavy 3rd generation quarks that would help us to understand why the Electroweak energy scale is where it is – i.e. why the Higgs mass is what it is and why the matter in the universe exists at the scale that we know. Beyond SUSY there will be many other searches for new particles and processes that have been proposed to resolve some of the same issues, or which are motivated by considerations of other symmetries, by string theory, or by considerations of additional dimensions and modifications to our understanding of gravity.
Q2/ Is it practically possible to find the most irreducible/ non material component of matter?
Whenever we enter a new, higher energy regime, we have the ability to probe smaller distance scales, simply because higher energies correspond to shorter wavelengths. We are probing distances as small as about 10^-19 to 10^-20 m ( known as a zeptometer) and we have not found any indications of substructure for particles we associate with matter, namely the quarks and the leptons (i.e. electrons, muons and tau’s). It is always possible that there is substructure and we just do not yet get to small enough distances to detect it. Certainly one cannot go below 10^-35 m, known as the Planck length, at which space-time breaks down. This is also the scale at which we could see particles as strings. It is a practical impossibility for us to probe to such a small scale as it would require an accelerator with a size of around 10^16 km. However, it is conceivable that we could detect things with much more reasonably sized accelerators that would have implications for much higher energy scales (much smaller distance scales). This is how we are now proceeding. We do what we can.
Q3/ What implications do this and further discoveries have in practical applications?
This area of research is pure physics, or what is referred to as “basic research”. If there were applications it would be applied physics. Indeed, there are no known practical applications for pure physics research but historically, deeper understanding of the laws of physics have ultimately led to major revolutions in technology. There are many standard examples from the past, like the direct application of quantum mechanics to chip design, and hence computers.
However, I do not believe that there are likely to be any such revolutions generated by the physics we are now uncovering. This is simply because we are studying particles that do not exist unless you build a machine like the LHC to produce them, and even then, they are very rare. Past breakthroughs in physics helped us to understand material at hand, so to speak, like atoms, then electrons and protons, and antimatter etc. What we are studying now is far more difficult “to find” and cannot be accumulated and stored. We discovered the Higgs boson, for instance, by observing an excess of several hundred proton-proton collisions in which we could reconstruct a particle with a mass of 125 GeV (around 133 times the proton mass). These several hundred collisions were extracted from a total of more than 1,000,000,000,000,000 proton-proton collisions produced over 2 years of LHC operation. So Higgs bosons can teach us a lot about the universe, but we cannot easily produce them and play with them. In fact, they decay in an amount of time that is shorter than we can even measure!
There are however many direct practical benefits arising as spin-offs of our research. As you probably know, CERN research is carried out in collaborations that span scores of nations. Coordinating such complex projects on a global scale like this required a new model of communication and sharing of information. This is why the World-Wide-Web was invented at CERN. More recently, we have developed a global computing network that allows any LHC researcher to launch data analysis jobs on any of 100s of thousands of processors spread across dozens of countries. This was necessitated by the large datasets (on the order of 10s of Petabytes) that are collected each year at the LHC. Development of such powerful global collaboration tools have had a huge impact on the world and our research continues to play a very high profile role in these areas (at a very tiny cost to taxpayers when compared to the benefits).
Q4/ Is it safe to make such seminal work so transparent?
Our studies are always public and in fact all LHC results are now published in open access journals. None of the results we are now obtaining have any potential for producing anything that could be considered dangerous. They inform us about the universe in which we live. They are cultural, timeless, and I believe that they enrich our human existence.
Q5/ Stephen Hawking’s comment, “… the Higgs Boson being the greatest threat…” – how much truth do you think it bears, if any at all?
This is a funny comment. Phase transitions occur in nature all the time, including such things as water turning to ice. The Brout-Englert-Higgs mechanism corresponds to a phase transition of the entire universe that occurred just a fraction of a second after the Big Bang. This is how and when the Higgs boson came into being. The Higgs field is everywhere in the universe and it is because of it that elementary particles have mass. For example, it is why electrons have mass, and without this mass we would not have atoms and so we would not exist. So it is ironic to say that the Higgs boson is a threat, since we would not be here without it. I believe that what is being referred to is the fact that the universe could undergo another phase transition that would cause the Higgs field to go away, which would be the end to all matter as we know it. So the threat is not due to the presence of the Higgs, but rather to the potential for it to go away.
(KATYAYANI CHOWDHRY IS A FREELANCE JOURNO/ AUTHOR. HER DEBUT TRILOGY IN SPECULATIVE FICTION, SHUNYATA- BEING FEMININE IS DIVINE, IS NOW AVAILABLE AT AMAZON AT THIS LINK: http://www.amazon.co.uk/SHUNYATA-BEING-FEMININE-IS-DIVINE-ebook/dp/B00VNSOBZI SHE CAN BE CONTACTED AT : katyayanichowdhry@gmail.com)